Channel Bonding In WiFi And Radio Frequency Physics
Using larger channels improves WLAN capacity, but the laws of RF physics can pose challenges when putting channel bonding into practice.
January 14, 2016
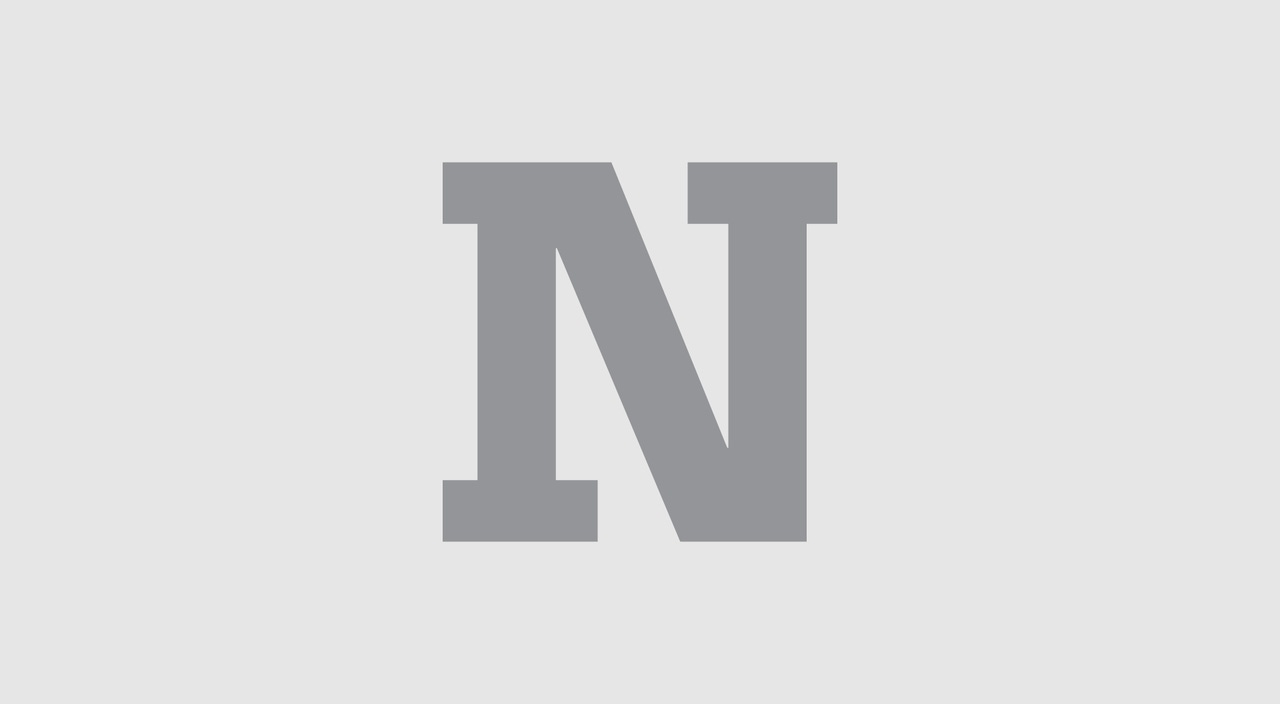
In the WiFi industry, we are on a never-ending quest always to push more and more data to more and more devices. A seemingly great mechanism for this is channel bonding, where neighboring 20 MHz channels are bonded together to form a larger channel. By doubling the channel width, you get slightly more than double the data capacity of the transmission.
Alas, however, as with all throughput enhancement techniques, channel bonding comes with a price. Implementing channel bonding in ever-increasingly complex WiFi networks is a careful balance of tradeoffs that can make it difficult for even experienced WiFi professionals to properly understand. The natural inclination is to make the channels as large as possible, and most access point vendors make it very easy to do this on their equipment.
However, what generally is not provided is guidance as to where and how larger channels should, and should not, be used. As a result, many modern WiFi deployments are problematic, because their environments cannot properly handle the use of larger channels.
In a series of blogs, I'll discuss the various tradeoffs involved in channel bonding, cover the regulatory restrictions, and provide guidance on where it should be used in WiFi deployments. In this first part, I'll discuss the immutable laws of radio frequency physics.
In radio communications, a receiver that hears multiple signals at the same time on the same (or overlapping) frequencies cannot distinguish between them. When this happens in WiFi, this is referred to as a collision. The multiple transmitters are causing co-channel interference (or adjacent-channel interference in the case of overlapping channels). The transmitted frame is corrupted, because the receiver cannot distinguish the intended frame from other signals on the same frequency.
In the WiFi protocol, a receiver sends an ACK to acknowledge proper receipt of the transmitted frame. If the transmitter does not receive the ACK, then it must assume a collision occurred and resend the same frame again (and again, and again) until the transmitter receives the acknowledgement. If a transmitter has to repeat the same frames over and over again, it diminishes the overall effective capacity of the channel.
When people complain about WiFi performance, it's a safe bet that quite a lot of collisions are occurring.
To provide contiguous coverage throughout a facility, some coverage overlap between neighboring access points is necessary. This can, however, create self-interference, where the APs within the same network cause co-channel interference and result in collisions for client devices in these overlap zones. To avoid self-interference, WiFi engineers can apply a process called channelization, which involves setting independent channels on neighboring access points and carefully controlling their individual transmission output powers so that their signals do not interfere with each other in these overlap areas.
Figure 1:
(Image: Pobytov/iStockphoto)
The ease or difficulty of channelization depends on the number of independent channels available. Since the size of the usable frequency band for WiFi per regulatory domain (i.e. country) is fixed, mathematics dictates that when the size of each independent channel is doubled, the number of available independent channels is halved, rounded down. Thus, as channel sizes get larger, channelization gets harder.
Larger channels also result in an increased noise floor, defined as the minimum threshold level below which a receiver cannot distinguish a signal from the background noise. There is naturally occurring background energy called thermal noise, as every object in the universe gives off very low levels of energy that are a function of temperature and bandwidth. For 20 MHz WiFi channels, this floor is approximately -101 dBm.
However, as channel sizes are doubled in size, the level of background energy also doubles (i.e. rises by 3 dB), increasing the noise floor to -98 dBm for 40 MHz channels, -95 dBm for 80 MHz channels, and -92 dBm for 160 MHz channels. Since the achievable data rate between two radios is a function of the signal-to-noise ratio (SNR) at the receiver, a higher noise floor results in lower effective maximum transmission speeds.
Most client devices, especially smartphones and tablets, have much weaker transmitters than the access points. Accordingly, a WiFi network really must be designed to maximize the signal strength of client devices, as received at the access point. The only practical way to increase SNR, therefore, is to minimize the distance and obstacles between the access point and the client devices. This, in conjunction with the growing need to support more and more client devices on the WiFi network, leads to deploying more access points to cover a given area.
Unfortunately, adding more access points can actually decrease the total capacity, since more access points and fewer channels makes channelization harder and self-interference more likely. Proper channelization of the access points becomes increasingly important. While counter-intuitive, larger deployments with more densely placed access points generally have higher total capacity with smaller channel sizes and proper channelization.
In my next blog, I'll discuss how the laws of RF physics intertwine with IEEE standards and worldwide regulatory domains to make the use of bonded channels challenging.
About the Author
You May Also Like